Subscribe to the blog
Advances in oligonucleotide therapeutics by molecular diversity
Jun 20, 2023 10:10:57 AM / The Bio-Works Team
Therapeutic oligonucleotides are relatively simple molecules in the world of molecular biology. They are single- or double-stranded segments of DNA or RNA of about 10–50 nucleotides. Yet, despite their simplicity, or even because of it, they are claiming a very tangible place in the list of new biological drugs.
The field of oligonucleotide therapeutics has been expanding swiftly over the past 20 years. Currently, they are being used against various diseases such as Huntington’s disease, Alzheimer’s disease, Duchenne muscular dystrophy, respiratory illnesses and coronary obstructive pulmonary diseases (COPD), asthma, diabetic retinopathy, and many cancers.
As more and more nucleic acid-based drugs are being developed and manufactured, purification methods for the downstream processing of biopharmaceuticals will be needed that can meet therapeutic demands. Current off-the-shelf products, originally developed for proteins, will need to be adapted or used with an mRNA, an siRNA, or a plasmid.
How do we handle the increasing diversity of chemical features?
We took a look at the variety of oligonucleotide therapeutics that are currently under development and what is behind this diversity.
Fomivirsen, the first antisense medicine with a 21-nucleotide modified phosphodiester backbone with a phosphorothioate linker, was licensed by the US FDA (United States Food and Drug Administration) in 1998 for the treatment of cytomegalovirus retinitis in AIDS patients.
Up to 2022, fifteen drugs based on oligonucleotide therapy had received regulatory approval from the FDA.
What are oligonucleotide therapeutics?
Oligonucleotide therapeutics are short strands of nucleic acid molecules (DNA or RNA) that can affect the expression of specific genes, proteins, or enzymes in the body. What makes oligonucleotide therapeutics unique is their ability to act directly on the genetic material, helping regulate gene expression and potentially cure the underlying cause of a disease ranging from congenital disorders to cancers. With ongoing technological advances, researchers are unlocking the full potential of these tiny molecular machines, paving the way for a new era of personalized medicine and effective treatment of human diseases.
What has led to this rapid growth of oligonucleotide drugs?
Oligonucleotide drugs can modulate a variety of targets, but in particular the more than 10,000 human proteins previously thought to be undruggable by small molecules or protein therapies. Identification of drug candidates requires only the identification of a target sequence on the RNA that is part of the disease pathogenesis.
The cost-effectiveness of designing, formulating, manufacturing, and purifying oligonucleotide drugs on an industrial scale is more efficient than that of small molecules. Simple and reliable manufacturing platforms are available, from batch plasmid bioprocessing and cell-free enzymatic synthesis to entirely chemical synthesis.
Many oligonucleotide drugs, including mRNA-based vaccines and cell therapies have good safety properties and fewer regulatory requirements, and the safety and efficacy of the drug molecule can be improved by making structural modifications.
One challenge for downstream processing is the sheer range of different types of oligonucleotide drugs
Antisense oligonucleotides
Antisense oligonucleotides (ASOs) are short single-stranded DNA or RNA molecules that bind to specific mRNA molecules and inhibit protein synthesis or affect splicing patterns by targeting pre-mRNAs. They are small, single-stranded (8–50 bases), and can modify RNA and impede protein expression. In 1998, the FDA approved the first antisense oligonucleotide Fomivirsen.
RNA interference
RNA interference is a mechanism that disrupts gene expression by inhibiting or degrading the mRNA.
RNAi includes two types of small RNA molecules known as small interfering RNA (siRNA) and micro RNA (miRNA) that play an essential role in gene regulation.
- The first siRNA to be approved was in 2018 and now there are 4 siRNA drugs on the market. siRNA are short non-coding, double-stranded RNAs that regulate gene expression by acting specifically on mRNA targets. The mechanism of siRNA interference is based on suppressing gene expression by mRNA degradation. siRNA consists of 19–21 nucleotides, with the TT and UU nucleotides largely overhanging at the 3′ end.
- miRNA are endogenously expressed, 21–25 nucleotides long single-stranded RNA fragments that play a crucial role in regulating gene expression. They break down (or upregulate) mRNA by acting on several targets and promote translational suppression, deadenylation, and decapping by attaching to a particular region in the 3′ UTR of the target mRNA.
CpG oligonucleotides
These are single-stranded, small oligonucleotides with unmethylated CpG dinucleotides in a specific area. CpG oligonucleotides act as a stimulant to immune system cells such as macrophages, B cells, dendritic cells, and monocytes that express Toll-like Receptor nine (TLR 9).
Aptamers
Aptamers are single-stranded RNA or DNA oligonucleotides or peptides that selectively bind to their target site with high specificity and affinity. Their activity is based on their potential to build three-dimensional structures with a target.
Although aptamers may be likened to antibodies in their interaction with the target, they surpass antibodies in their target specificity, synthesis, and cost-effectiveness. Their superior features include good stability, longer shelf life, and large-scale high-speed production while maintaining high reproducibility and reliability. They are, for example, used as adjuvants together with cancer drugs in clinical trials.
Decoys
Decoys are single-stranded RNA oligonucleotides that target transcription factors and consist of a 2′-O-methyl alteration in the ribose sugar moieties of the nucleotide strand. This confers stability, and they are a powerful tool in gene therapy. Presently, decoy-based oligonucleotides for use in auto-immune and inflammatory diseases are in Phase III clinical trial stages in Japan and the United States.
Great therapeutic potential but there are challenges with oligonucleotides
Despite their great therapeutic potential, there are several limitations to oligonucleotide therapeutics. The major problems are toxicity, drug delivery, and uptake.
Another problem is degradation by nucleases, particularly of natural oligonucleotides. Double-stranded RNAs and naked siRNAs are degraded or fail to enter the cells and are rapidly cleared from circulation even before they show any activity.
Specific and efficient delivery of the oligonucleotide to the target tissues is challenging, primarily through the blood-brain barrier (in neurodegenerative diseases).
Two main strategies to overcome the challenges of oligonucleotides
Uptake and delivery of oligonucleotide drugs remain the most significant challenges. They need to travel through the bloodstream, pass through biological membranes, and be taken up by cells. They must avoid lysosomal and enzymatic degradation and bypass entrapment in secretory vesicles.
A couple of strategies are being widely used to enhance the stability of oligonucleotide drugs and promote delivery to target tissues.
Chemical modification strategies
An effective way to improve oligonucleotide drugs is to modify the sugar or make changes in the phosphate backbone.
In first-generation oligonucleotides, a sulphur atom replaced an oxygen atom in the phosphate backbone to impart resistance to endonucleases and improve bioavailability – producing the phosphorothioate oligonucleotides. This change, however, not only diminishes the affinity for the target but also introduces toxicity.
2'-O-methoxyethyl (2'-MOE) oligonucleotides: 2'-MOE oligonucleotides contain a methoxyethyl group at the 2' position of the ribose sugar. This increases the stability of the oligonucleotide and improves its affinity for RNA molecules.
Conformationally restricted DNA analogs like tricyclo-DNA (tcDNA) and locked nucleic acid (LNA) tend to have increased stability and enhanced affinity for RNA molecules. LNA with a methyl bridge between the 2′-O and 4′ carbon positions of the ribose ring, and tcDNA with an ethylene bridge and a cyclopropane ring between the 3′ and 5′ carbon positions of the ribose sugar.
Third-generation oligonucleotides involve modifications of the nitrogenous bases that form the oligonucleotide polymer. For example, phosphorodiamidate morpholino-oligomers (PMO) have a morpholine ring with phosphorodiamidate attachments in place of the nucleic acid backbone and peptide nucleic acid (PNA), whose bases are linked by amide bonds instead of phosphodiester bonds. Both these oligomers are chargeless, resistant to degradation by nucleases, and exhibit variable affinity for the target RNA sequence.
Conjugation and targeting
Another way to improve the drug properties of the oligonucleotide is to conjugate it to other molecules such as:
- Polymers such as Polyethylene glycol (PEG), a flexible, uncharged, hydrophilic polymer that protects the conjugated drug from nuclease degradation by forming a hydration shell.
- N-acetylgalactosamine (GalNAc). The GalNAc moiety enhances the uptake of the oligonucleotide by hepatocytes, improving its efficacy in treating liver diseases.
- Antibody conjugates are used as a carrier for many oligonucleotide therapies.
- Lipids such as cholesterol, in Lipid Nanoparticles (LNPs) that can enhance endosomal release, resulting in longer plasma half-life.
- Peptides, usually short cationic or amphipathic peptides (less than 30 amino acids in length) that can translocate oligonucleotide drugs across various biological barriers.
- Receptor ligands for tissue-specific targeting.
- Aptamers that are easy and cost-effective to design and conformationally flexible, have low immunogenicity, confer nuclease resistance and improve blood plasma half-life.
These two strategies alone are resulting in an enormous increase in the diversity of new purification targets.
The preferred method of oligonucleotide purification
For a long time, biopharmaceuticals were mostly various forms of proteins, and downstream purification protocols were developed to cover all the relevant parameters for these types of molecules. These new forms of oligo-based drugs and other types of promising new drug modalities, such as modified peptides, can have very different physiochemical properties.
Until new processes and tools for downstream purification can be developed, drug developers are forced to begin by testing the off-the-shelf purification products, the majority Ion Pair chromatography (IPC) or Anion Exchange chromatography (AIEX), and trying to adapt them for use with a unique molecule.
To address these challenges, new purification methods will need to be developed, but in the meantime, a solution could be to customize an existing chromatography product that can meet your oligonucleotide purification needs.
For example, with experience of creating and modifying chromatography resins, it is possible to modify several parameters of the resins using knowledge of how each can affect the final results.
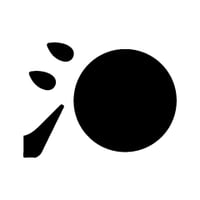